In the July issue of The O&P EDGE, I discussed the traditional phases of gait and major motions in the sagittal plane. However, the act of walking occurs in three-dimensional space, and it is well understood that body motion in all three planes is highly interdependent. Retracing the full cycle, we will now consider more subtle but equally important motion in the transverse and frontal planes. As an evolutionary adaption of bipedal locomotion, the tarsus of the human foot is a critical transformer of motion across all three planes.
Synergy of Motion
Generally speaking, the objective of walking is to make forward progress in a straight line. It also appears that, absent other factors, a second aim is to achieve this motion using the minimal amount of energy per step. Although walking primarily entails the action of the lower limbs, in order to remain smooth, it requires the coordination of the entire body. Arm swing and shoulder counter-rotation are employed to ensure an efficient stride. In traditional gait analysis, the body is often divided into two segments. The upper body, comprising head, arms, and trunk (HAT), is considered a passenger sitting atop the locomotor-the legs and feet. The pelvis plays a unique role at the junction of these two units and is essential to dampening abrupt changes in the movement of the center of mass.
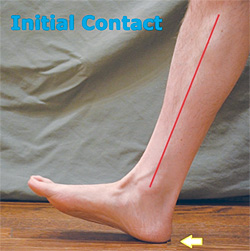
Initial double stance starts at the moment of contact or heel strike and lasts until mid stance. The effect of the ground reaction force (GRF) pronates the subtalar joint (STJ), and this in turn unlocks the midtarsal joint (MTJ). The foot then actively pronates as the tibia rotates internally. The descending lateral side of the foot loads, and the first ray plantarflexes gently, bringing the forefoot to the ground. This is a critical event in the cycle, and controlled pronation is necessary to facilitate internal tibial rotation and shock absorption. In addition, the mildly everted foot is more stable. If the foot fails to pronate at this early stage, there is the potential for ankle sprains, stress fractures of the lesser metatarsals, and pain along the lateral column of the foot.
The Role of Orthotics
Orthotics are helpful in directing GRF and motion, and extrinsic posting provides a stable platform for the rearfoot. Typically, an extrinsic medial post or an intrinsic Kirby heel skive is prescribed for an everted foot. The extra-high walls of a University of California Biomechanics Lab (UCBL) orthosis can also help guide calcaneal position, although patients with a rigid foot type may not tolerate this device. Pedorthic interventions such as sole wedges and wide flares on shoes can stabilize ankle position.
As the patient moves through mid stance, pronation of the STJ proceeds, forefoot loading is complete, and the foot is plantigrade. Orthotics can play a vital role in controlling mid-foot drop and avoiding the tissue stresses associated with reaching the end of the range of motion (ROM). Full body weight now moves over the foot, and due to the dynamic forces, this is one of the two moments in the cycle when GRF actually exceeds body weight. Common pathologies associated with excessive pronation include plantar fasciitis, heel pain, and posterior tibial tendon dysfunction (PTTD). A well-fitting custom orthotic will support the medial longitudinal arch (MLA), thus helping to maintain the structural integrity of the foot.
In the transition from mid stance to terminal stance, still in single-limb support, the body weight is now aligned over the forefoot and heel rise begins. This starts a reversal in the chain of events that occurred at initial contact. As the swing leg is moving forward and rotating the pelvis, the supporting leg naturally rotates externally. As the center of pressure moves forward and heel rise continues, it is critical to have motion at the first metatarsal phalangeal joint (MPJ). Clinical studies show that a dorsiflexed first metatarsal restricts motion and jams the first MPJ. When this occurs in weight bearing, it is called a functional hallux limitus. Orthotics that plantarflex the first metatarsal help combat this. By requesting minimal plaster arch fill to raise the longitudinal arch and adding a reverse Morton’s extension, you may be able to restore some of the lost ROM.
The windlass mechanism is engaged due to the dorsiflexion of the hallux. The mechanical effect is to pull the plantar fascia taut and close-pack the bones of the MLA, forming the rigid lever essential for propulsion. The natural mortises of the foot bones re-mesh, the MTJ locks, and the foot becomes stable. Any interruption in this sequence of events can be disastrous as it will leave the foot structure “open” and subject to severely deforming forces. The most severe cases of adult-acquired flatfoot and PTTD are associated with feet that continue pronation late in the gait cycle, missing the appropriate window for resupination. The efficacy of foot orthotics lies in their ability to both enhance foot architecture and alter the timing and duration of key events.
Considering the entire cycle, the presence of an asymmetry will often lead to dysfunction. It may be the result of a leg length discrepancy (LLD), joint restriction, pain, or another imbalance. The effect is unequal loading, which could eventually lead to overuse or uneven wear. For example, there are several compensations for LLD including extended contact time and lowering of the MLA on the longer limb. Conversely, one might see the MLA rise on the short limb. Finally, there may be an altered step length or a change in ROM at the ankle, knee, or hip.
There is far more to appreciate about the complexity and synchronicity of gait, and this article serves merely as an introduction. As the body gracefully moves over the ground, the delicate dance and transfer of body weight occurs in fractions of a second. With an understanding of normal walking, we can begin to tease out deviations that may be the cause of pathology.
MOVEMENT COUPLING: TRANSVERSE MOTION
TRANSLATED TO THE FRONTAL PLANE
When the foot hits the ground at initial contact, as seen from above, the pelvis is at an angle of 4 to 5 degrees in the transverse plane. Within the course of the next step, as the swinging leg propels forward, the pelvis rotates forward 8 to 10 degrees. Due to compounding effects, the stationary weight bearing leg goes through a magnified rotation, with both the femur and the lower leg rotating externally. If the foot were simply a solid block, straight hinged to the body at the ankle, this would force the foot to torque outward rather significantly with each step. In order to remain firm on the ground without slipping externally, the foot is able to accept the transverse rotation of the leg above the ankle and translate it into frontal plane motion below. This remarkable engineering feat is achieved by a number of mechanisms working in concert.
At heel strike, when the STJ pronates, the foot everts and the calcaneus shifts laterally. This has the effect of allowing the talus to angle down. The altered orientation of the calcaneus and talus facilitates two movements. First, the MTJ unlocks and opens, allowing motion along the longitudinal axis of the foot. Second, with the talus lowered, the tibia rotates internally. There is a clear sequence of STJ pronation (motion in the frontal plane) correlating to internal tibial rotation (motion in the transverse plane). This interlinked motion between the tibia and calcaneus is sometimes referred to as movement coupling and is beautifully illustrated in diagrams from Inman et al.’s classic text, Human Walking.1 These schematics show that the integrated action of the lower leg and foot can be conceived as being similar to a universal joint or beveled gear (Figures 1a and b).
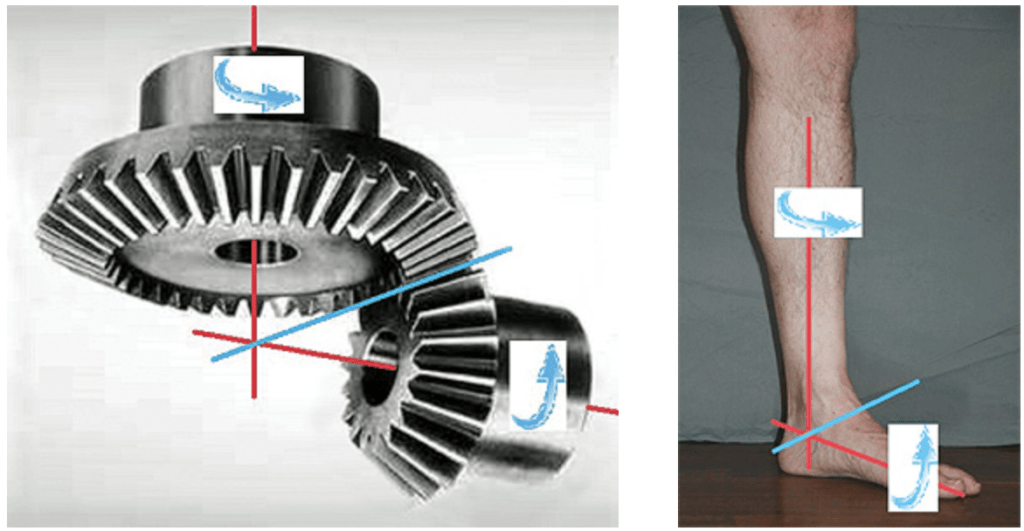
Figures 1a and b: The mechanism of the STJ and movement coupling compares to a bevel gear.
Internal rotation helps the tibia move more easily over the fixed foot, as the oblique axis of the ankle joint decreases. Later in the cycle, the sequence is reversed. The swinging limb pulls the pelvis forward, which externally rotates the supporting leg, allowing the foot to invert and regain stability.
The author gratefully acknowledges the help and contribution of Paul R. Scherer, DPM, MS, clinical professor at Western University School of Health Sciences, College of Podiatric Medicine, and founder of ProLab Orthotics, Napa, California.
References
- Inman, V. T, H. Ralston, and F. Todd. 1981. Human Walking. Baltimore, London: Williams & Wilkins.