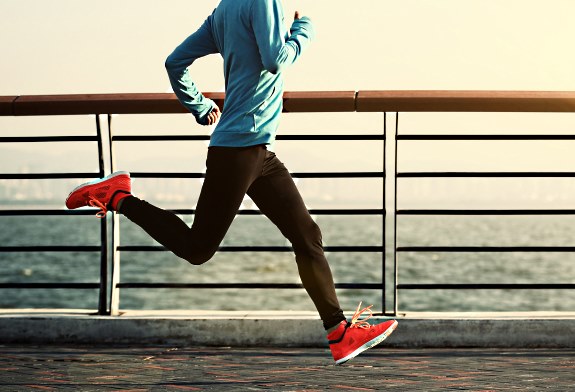
Courtesy of Shutterstock Inc.
In the late 1870s the eccentric Eadweard Muybridge began pioneering work photographing bodies in motion. His iconic pictures of people and animals opened a new chapter in understanding movement and the human body. Walking and running, similar insofar as they are bipedal activities, are quite distinct from a biomechanical perspective. As a profession, we spend much of our time evaluating normal walking gait. Detailed studies and research have been conducted in this arena, but biomechanists have also extensively studied running. There is an interesting body of work on this complex activity. This article looks at some ways in which running is unique and should be considered separately.
Running Biomechanics Cycle
Walking has been referred to as the art of controlled falling. It can be characterized as moving the body’s center of mass (COM) forward and then catching it with the leading leg. Hardly a full description of gait, this captures the notion that when walking, we are moving between equilibriums. One foot is always in contact with the ground, and for a significant portion of the full cycle, some part of both feet are on the ground. This is known as double stance. In some of Inman’s diagrams of ambulation, gait is represented as two legs of a divider-compass swiveling forward, the body wobbling between points of near equilibrium.1 More recent models liken walking to the action of an inverted pendulum, with the body moving over a fixed foot, somewhat like the motion of a metronome.
In contrast, running begins when there is some period of no ground contact. Double float is the term used to describe the time when neither foot is touching the ground. Stance phase is less than 50 percent of the overall cycle. There are two periods of double float, when both limbs are off the ground, and there is no time when both feet are on the ground simultaneously. The faster the speed, the shorter the stance phase and the more time the runner spends airborne.
Initial contact occurs when the forward foot makes contact with the ground. Based on running style and speed, the hind foot, midfoot, or forefoot may strike first. In preparation for this contact, COM has been falling and the body has been decelerating. Through this absorption (braking) period, the knee and ankle flex to dissipate energy and to store it in muscles and tendons. While still in stance phase, a clear reversal occurs as the body prepares for propulsion. Stance phase generation is marked by the ankle, knee, and hip extending, with the leg muscles and tendons returning some of the previously stored energy. The COM moves upward and forward in preparation for toe-off (Figure 1).
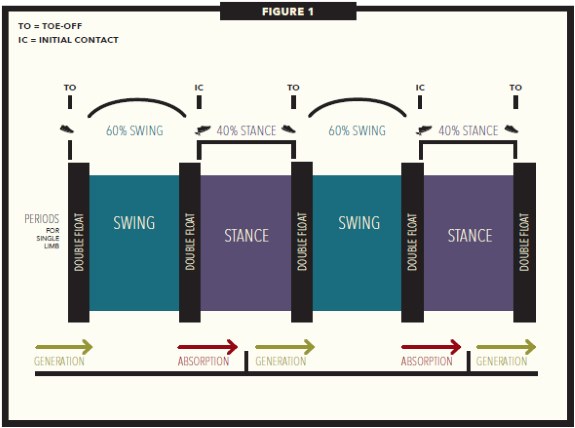
Muscle-tendon Elasticity
Studies conducted on the metabolic cost of running reveal that energy consumption is lower than predicted. It became clear that bodies do not move simply due to muscular activity. Both in walking and running, we are not just pushing ourselves forward. With this insight, researchers began to investigate and understand the mechanisms that lead to efficient running. From animal studies, scientists understand that muscles and tendons have elastic properties that allow them to temporarily store energy. As an analogy, consider a bouncing rubber ball. It is continually converting the potential energy, at its high point, into kinetic energy, just before it hits the pavement. Likewise, a runner’s potential energy is highest when in double float and lowest at mid-stance. The body uses gravity during absorption to load the muscle-tendon storage springs and then returns this energy in the second half of stance in preparation for toe-off.
EMG plots of muscle activity show that muscles fire in anticipation of initial contact so they can act as tensioners for the tendons. This is also known as the stretch-shortening cycle, where muscles work eccentrically prior to concentric activity. During the absorption phase, the muscles act eccentrically as the tendons stretch. Later, during generation, the muscles contract and the tendons recoil. Both the higher step cadence of running and the deforming force of gravity allow for the efficient, temporary storage of elastic energy that is reused for propulsion. Elasticity of ligaments in the arch of the foot also contribute to this phenomenon. It appears that these mechanisms save approximately 50 percent of the energy that would otherwise be required.
Stride Length and Frequency
It would seem intuitive that physical body dimensions such as height, leg length, and limb mass would be predictors of stride length and other running variables, but this does not appear to be the case. One stride, a full cycle, is from initial contact to the next contact of the same foot. Although there is some correlation that taller and longer-legged individuals take longer strides, a runner’s optimal stride length is far from predictable.
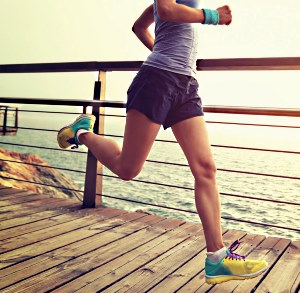
Courtesy of Shutterstock Inc.
In general, we increase both stride length (distance, m) and stride frequency (strides per second, Hz) as speed increases. At a given running speed, short of sprinting, most individuals choose their stride length and stride frequency to minimize metabolic energy cost. Many variables will determine the right combination of these two, including speed, gradient, running surface, and body dimensions and proportions. Runners tested over a range of velocities (3m/s to 4m/s, an increase of 33 percent) increased their stride length, on average, by 28 percent, while stride frequency increased by only 4 percent. This indicates that most runners have a fairly narrow band of preferred stride frequency when distance running.
It has been proposed that this preferred stride frequency is chosen subconsciously as the most economical for an individual runner, although it is possible to adjust. The period from initial contact to mid-stance acts as a brake on the runner and should be minimized. Several well-known running techniques, including the Pose Method® and ChiRunning, advocate a faster stride to minimize braking and capitalize on the energy-return effect. Underscoring this, a recent study demonstrates that trained runners have a higher step rate and shorter step length than untrained runners moving at the same speed. The trained runners are also about 7 percent more efficient. It appears that by optimizing stride frequency, running performance can be improved for some individuals.
Arm Swing
In normal walking, arm swing plays a part in maintaining a balanced and efficient gait. Biomechanists developed the concept of counterrotation to explain how upper body and arm swing work opposite leg swing. In walking, arm swing helps to reduce vertical oscillations of the COM, whereas in running, it contributes to vertical motion.
Researchers studied the role of the arms in aiding propulsion (forward) and lift (vertical). Essentially, the arms do not contribute to forward motion. This is clear when you consider the arms oppose each other and the forward momentum of one side will cancel that of the other. However, isolating the COM of the arms from the rest of the body, it is seen that the arms can play a role in providing lift. As running involves an airborne phase, this contribution to lift is helpful. As speed increases, arm swing becomes more important and arm motion becomes more vigorous and animated.
The arm movement pattern is opposite that of the legs and this assists in smoothing out changes in total body momentum. As the right leg comes forward through swing, the left arm also moves forward. This synchronous motion of opposing limbs is essential to keep the body’s COM moving smoothly in a forward direction. Although arm motion cancels itself out in the forward direction (sagittal plane), this is not the case when considered through the vertical axis (transverse plane). Seen from above, with the left arm coming forward and the right arm going backward, they are both contributing to the same angular momentum. In this example, the whole upper body is twisting in a clockwise direction. This occurs as the lower body rotates counterclockwise. The contribution of the arms and upper trunk nearly cancel out that of the lower trunk and legs. This is a neat solution to the fact that the runner spends portions of each swing phase airborne. Without this cancelling of angular momentum, the leg in swing phase, during double float, would twist the entire body while it is in the air. Running would then become a series of overcorrecting, inefficient leaps approximating a straight line.
Potential and Kinetic Energy
Within the gait cycle there is an interplay of potential and kinetic energy. In walking, the potential energy is highest at mid-stance while the kinetic energy is lowest. The potential and kinetic energies are out of phase, swapping off through each step. During running, the energies are in phase working together to propel the body into double float.
One of the subtle distinctions between walking and running is the relative height of the COM at mid-stance. In walking, the COM is highest at mid-stance, as the body “vaults” over the fixed leg. Conversely, in running, the COM is lowest at mid-stance, as the body prepares to push itself forward. It is an interesting difference that highlights the alternate uses of available energy.
The principles referred to in this article mainly relate to distance running and maintaining aerobic metabolic efficiency. Sprinting is different in that it does not seek economy but rather maximizes power output. Sprinters have a different strike pattern and tempo, and their muscles work anaerobically in the short term to gain speed over efficiency. Running is a fascinating choreography of the body with intricate patterns of repetitive movement and transformation of energies. It is a wonderful, graceful coordination of body movement. This serves only as an introduction to the topic, an entryway to further study and understanding. Interested readers are encouraged to pursue the references listed.
References
-
- Inman, Verne Thompson, Henry James Ralston, and Frank Todd. 1981. Human walking. Baltimore, London:Williams & Wilkins.
- Cavanagh, P. R., ed. 1990. Biomechanics of distance running. Human Kinetics Publishing.
- Novacheck, T. F. 1997. The biomechanics of running. Gait & Posture 7 (1):77-95.
- Perry, J. and J. Burnfield. 2010. Gait analysis: Normal and pathological function. Thorofare, NJ: Slack.